Development of an experimental database of emission spectra from various elements using LHD
Various elements with atomic numbers from 36 to 83 have been injected into LHD plasmas to observe their emission spectra. Spectra for various atomic numbers and electron temperatures have been systematically investigated by comparisons with theoretical calculations. As a result, some spectral lines have been newly identified. The present study has contributed to the development of an experimental database, useful not only for fusion, but also for atomic physics and plasma applications.
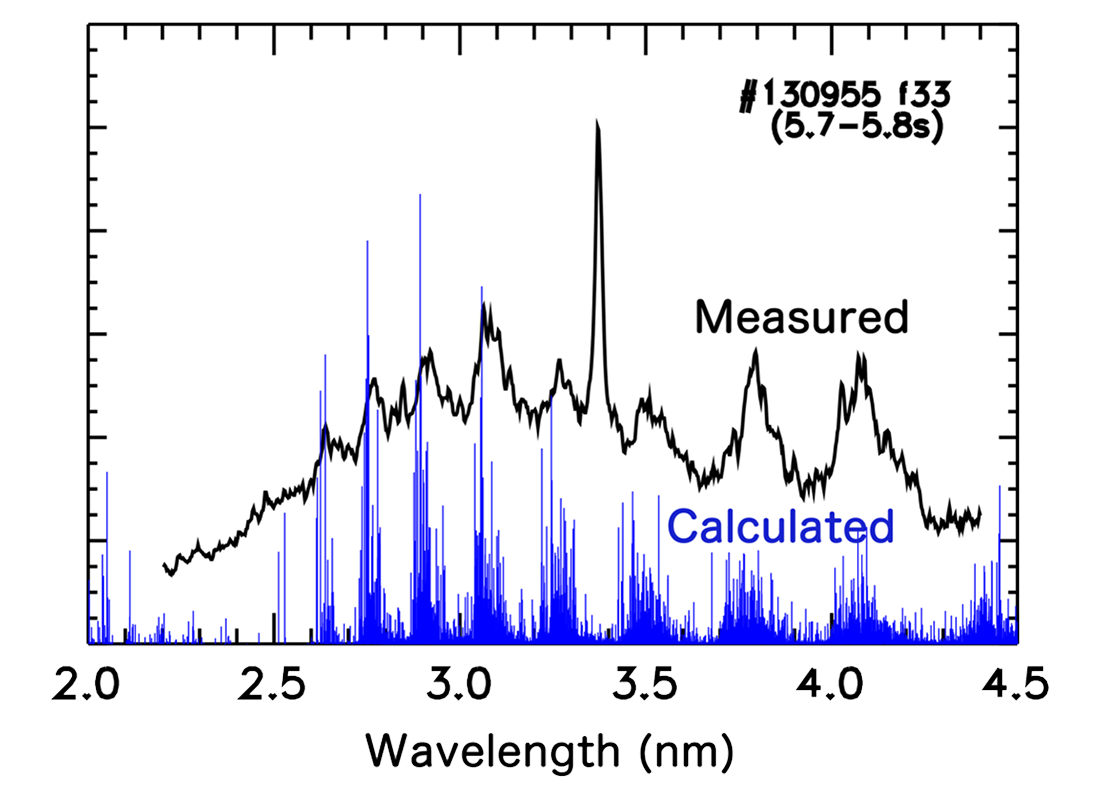
Various elements with atomic numbers from 36 to 83 have been injected into LHD plasmas to observe their emission spectra. Spectra for various atomic numbers and electron temperatures have been systematically investigated by comparisons with theoretical calculations. As a result, some spectral lines have been newly identified. The present study has contributed to the development of an experimental database, useful not only for fusion, but also for atomic physics and plasma applications.
Plasmas produced in the LHD can be regarded as a light source which has unique characteristics such as high temperature, high emissivity and good stability. In addition, the LHD is equipped with impurity injection systems and advanced diagnostic systems. Therefore, the LHD can be utilized for spectroscopic studies on various elements. In this study, we injected more than 20 elements, the atomic number of which were chosen in the range of 36-83, into LHD plasmas to systematically observe emission spectra in the extreme ultraviolet and soft X-ray energy regions. Some of these elements are important in fusion or industrial applications, or have not been measured previously.
In the LHD experiment, we introduced impurities into stable hydrogen plasmas generated by neutral beam injection heating, using a tracer encapsulated solid pellet or gas puffing. Emission spectra in the wavelength range of 1-20 nm have been recorded by a grazing incidence spectrometer with a frame rate of 10 Hz. Temperature dependence of the spectra can be measured in a wide range by intentionally forming a "temperature hole", in which the electron temperature is zero at the center. The "temperature hole" was generated by controlling the heating power and the amount of injected impurities.
Impurities in high temperature plasmas exist as highly charged ions in which a number of electrons are stripped away. One can suppose that electrons are distributed in annular layers around a nucleus called a K-shell, L-shell, M-shell, etc. from inner to outer layers. When an electron changes its energy state, it emits a photon with a wavelength inversely proportional to the energy difference between its initial and final states. Because the energy level structure depends on an element and an electron configuration, the resulting emission spectra have structures specific to them.
Under the plasma conditions in the LHD, the major emitters are ions with M-shell outermost electrons (M-shell ions) for elements with atomic numbers approximately from 30 to 50, and ions with N-shell outermost electrons (N-shell ions) for elements with atomic numbers approximately higher than 50. The spectral feature of N-shell ions drastically changes as the electron temperature changes. Namely, simple spectra composed of a smaller number of spectral lines in high temperature conditions change into complex dense spectra, composed of a huge number of spectral lines in low temperature conditions. In this study, we found some spectral lines which have not been experimentally identified, based on the analyses of atomic number dependence and comparisons with theoretical calculations.
For either M-shell or N-shell ions, the spectral feature depends on whether or not the jumping electron changes its shell. If the jumping electron does not change its shell (Δn=0 transition), spectra from a wide range of charge states are superimposed around a specific wavelength. On the other hand, spectra from different charge states tend to be separated if the jumping electron changes its shell (Δn≠0 transition). As a result of comparisons between the measurements and the calculations, it was revealed that the calculated wavelengths tend to be systematically shifted to shorter wavelengths for the Δn=0 transitions, while they are in good agreement for the Δn≠0 transitions, in which spectral structure can be clearly linked to the associated ion stages.
From the viewpoint of atomic physics, the above results would be useful in a fundamental database for improving spectral simulations for heavy ions. Besides, they can be applied to spectral analyses of tungsten, one of the plasma facing components in the ITER tokamak, as well as candidate elements for next generation semiconductor lithography light sources.
This study was carried out by C. Suzuki, I. Murakami, et al. (NIFS), in collaboration with F. Koike (Sophia Univ.), T. Higashiguchi (Utsunomiya Univ.) and G. O'Sullivan (University College Dublin).
This research result was published in X-ray Spectrometry, a journal on the theory and application of x-ray spectrometry, on December 26, 2019.