Measurement of ultraviolet emissions steadily confirms powder drop into plasma
In the Large Helical Device (LHD), boron powders are dropped into the plasma to form a boron layer on the wall of the plasma confinement vessel. Whether the dropped boron reaches the plasma efficiently or not can be confirmed by spectroscopic measurement of the emission of boron ions in the plasma. In particular, by measuring "ultraviolet" light, which has a shorter wavelength than "visible light" that we can see, we can simultaneously observe the emission of boron ions from low to high charge states.
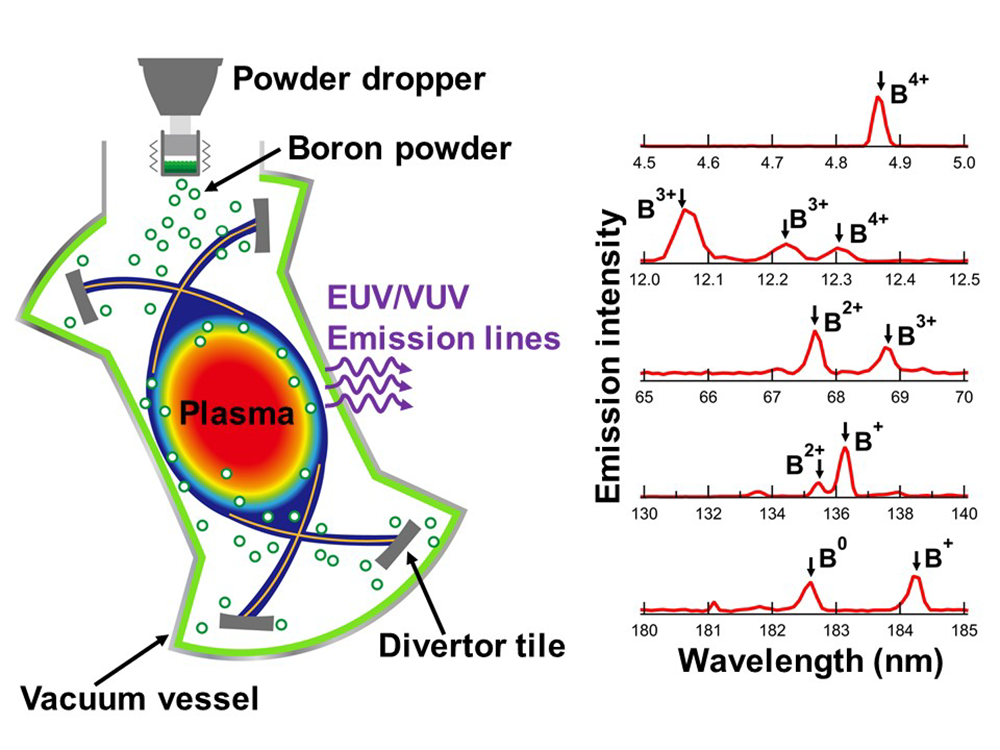
In order to realize nuclear fusion power generation, hydrogen plasma with a high temperature of over 100 million degrees Celsius must be stably confined in a magnetic field. In addition to hydrogen, the plasma contains impurities such as carbon, oxygen, metals, and other atoms and ions emitted from the walls of the plasma confinement vessel and the heat-receiver called the divertor plate. Impurity ions absorb energy from the plasma and emit it as light, lowering the plasma temperature. In other words, to keep the plasma temperature high, impurities must be reduced.
One way to reduce impurities in the plasma is to create a thin layer on the walls of the plasma confinement vessel to suppress the release of impurities from the walls. In the Large Helical Device (LHD) at the National Institute for Fusion Science, a layer of boron (atomic number 5, element symbol B) is made on the walls before the start of each annual experiment. In addition to this, a device that drops boron powder into the LHD plasma has been installed with the aim of simultaneously forming a boron layer on the wall while conducting plasma experiments. This powder dropper has been installed since the 21st campaign of LHD experiments (October 2019-February 2020) under an international collaboration between the National Institute for Fusion Science and the Princeton Plasma Physics Laboratory in the United States.
For a successful experiment using the powder dropper, it is necessary to confirm that the dropped powder reaches the plasma efficiently. What is useful here is a technique called "spectroscopy," which measures the light emitted by the plasma by dividing it into different wavelengths. Ions in plasma emit light at different wavelengths depending on their species. This is called the "line spectrum" of the ions. By examining the wavelength and light intensity of this line spectrum, the species and amount of ions can be determined. In an experiment in which boron powder is dropped, the observation of the line spectrum of boron ions is evidence that the boron powder has reached the plasma.
The plasma in the LHD has an electron temperature of several tens of millions to 100 million degrees Celsius near the center and several hundred thousand to several million degrees Celsius at the edge. Ions in plasma in this temperature range strongly emit ultraviolet light, which has a shorter wavelength than visible light. Ultraviolet light is further classified into Extreme Ultraviolet (EUV), which has a shorter wavelength, and Vacuum Ultraviolet (VUV), which has a longer wavelength. The LHD constantly measures spectra in the EUV region (0.5 to 30 nm) and the VUV region (30 to 240 nm). We investigated the EUV and VUV wavelength spectra when boron powder was dropped, and summarized what kind of line spectra were observed. As a result, we found that line spectra of boron from neutral atoms (B0) to tetravalent ions (B4+) can be observed simultaneously, as shown in the figure. When the powder type was changed to boron nitride, a compound of boron and nitrogen (atomic number 7, element symbol N), there were line spectra of nitrogen divalent (N2+) to hexavalent (N6+) in addition to the line spectrum of boron. In general, ions with lower charge states exist in regions of the plasma with lower temperatures, while ions with higher charge states exist in regions of the plasma with higher temperatures. Therefore, this observation provides evidence that the dropped powder has definitely reached the plasma and that the ionized ions are distributed over a wide temperature range in the plasma.
Line spectra, which are easy to measure due to their large emission intensity, are also useful as rough indicators of ion abundance. For example, the line spectra of tetravalent ions at a wavelength of 4.9 nm and of monovalent ions (B+) at a wavelength of 136.2 nm are indicators of the amount of boron ions inside and outside the plasma confinement region, respectively. By providing such line spectrum data for all powder drop experiments and constantly checking how strongly boron ions are emitting in the plasma, it is now possible to efficiently conduct experiments while adjusting plasma conditions and the amount of powder dropped for each discharge.
This research was conducted by a research group consisting of Assistant Professor Tetsutarou Oishi, Associate Professor Naoko Ashikawa, Professor Suguru Masuzaki, Associate Professor Mamoru Shoji and others at the National Institute for Fusion Science, in collaboration with Dr. Federico Nespoli, Dr. Eric P. Gilson, Dr. Robert Lunsford and others at the Princeton Plasma Physics Laboratory in the United States, which is supported by LHD-PPPL collaboration.
The research results were presented as an oral presentation at the third workshop (September 23 and 24, 2020) of the Bilateral Joint Research Project " Control of wall recycling on metallic plasma facing materials in fusion reactor" (April 1, 2019 - March 31, 2023), which is supported by the Japan Society for the Promotion of Science (JSPS) and the Chinese Academy of Sciences (CAS). The presentation was highly appreciated by researchers in both Japan and China. Based on the discussions there, a paper describing the experimental results in detail was published in "Plasma Science and Technology," an international journal on plasma science and technology, on June 30, 2021, and was also selected as one of the journal's 2021 highlight papers.