Cooling a plasma of 100 million degrees by ice pellet – Emergency plasma shutdown system in ITER
The Large Helical Device has performed a new experiment that injected ice pellets made of neon, which are planned to be used by the emergency shutdown system in ITER. To mitigate or avoid the damage of the plasma facing components, ITER will inject ice of hydrogen and neon to cool down the plasma when a precursor of the plasma instability is observed. In the present experiment, we successfully observed the behavior of the injected neon pellets ablating in a high temperature plasma.
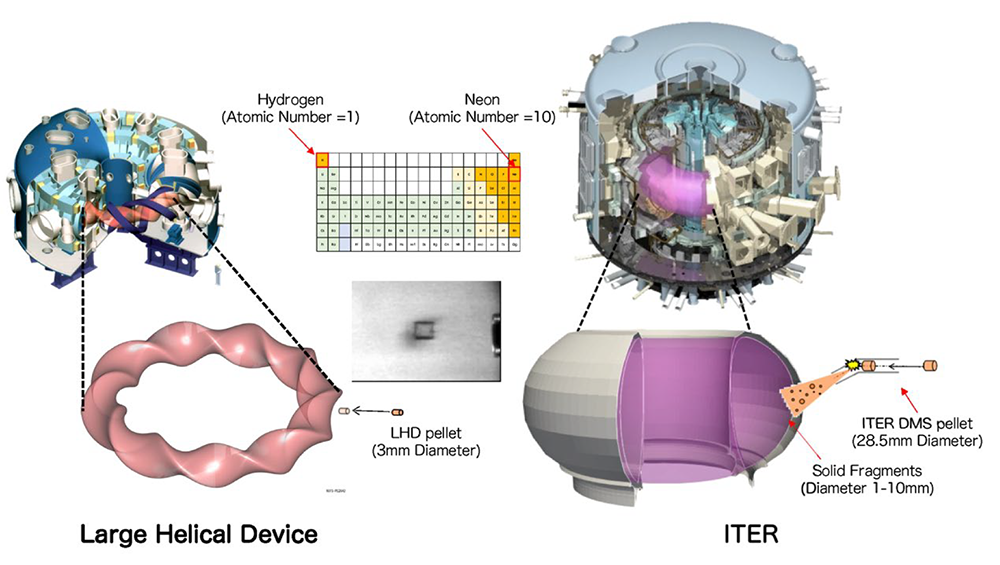
In a fusion power plant, a fusion reaction between deuterium and tritium is maintained by heating of fuel to a temperature of 100 million degrees. Because there is no material that can withstand such a high temperature, the fusion core plasma is controlled using a magnetic field structure, so that it does not touch the material wall. ITER (“The Way” in Latin) is now under construction at Saint-Paul-lez-Durance (France) through international collaboration including that of Japan. It will be the first fusion device to produce net energy.
ITER will tackle many challenges in operation of the largest magnetic fusion device in history. To achieve the mission goal, therefore, exceptional handling is the most important function of the device. A major concern is “disruption”, which is an event such that the magnetic configuration to confine the plasma is distorted by major instabilities. The plasma energy then flows onto the surface of the material wall within a short time scale of a few milliseconds. The heat and electromagnetic loads associated with a disruption may damage the in-vessel components and may severely affect the operation cycle and the machine lifetime of ITER.
To mitigate such a risk, the development of a rapid shutdown system has been a key research area in last twenty years. Recently, ITER has made a decision on the baseline concept of the scheme applied to ITER – “Shattered Pellet Injection (SPI)”. In this scheme, when a precursor of the plasma instability is diagnosed, the control system will inject ice made of a mixture of hydrogen and neon to cool down the core plasma before it damages the surrounding material.
Subsequently, when the core heating was turned off, the relationship between the temperature gradient and the driven heat flux, as the temperature profile reverted from a peaked profile to a hollow one, was investigated. It was found that the trajectory was different from that when the core heating was applied. This phenomenon is called transport hysteresis. We found that the transport at a certain location is not determined by the temperature gradient or temperature at that location. We observed that this hysteresis was repeated by turning the core heating on and off. The non-local nature of non-diffusive heat transport, associated with heat convection, was experimentally revealed as the electron temperature moved back and forth between the states of peaked, flat, and hollow profiles. This finding is a new insight into transport phenomena in fusion plasmas.
Because the reliability of this system is of critical importance for the operation of ITER, we need to know the cooling process of the pellets in as much detail as possible. To contribute to the R&D of ITER SPI, the Large Helical Device (LHD) performed a new experiment that injected ice pellets made of neon. The LHD has operated the injector of hydrogen ice based on a similar system that will be used by ITER SPI for more than twenty years. In addition, world-leading diagnostic systems and high repetition rates of plasma pulses of the LHD offer a good platform to study the interaction of pellet ice with a high temperature target plasma.
We recently reported the initial results of pure neon pellet injection into the LHD plasma at the International Toki Conference (ITC30) in November.
We used the diagnostic signals that were observed at a different position of the donut structure of the LHD to estimate the expansion speed of ablated materials. We have shown that the ablated material from neon expands more slowly than that from a pure deuterium pellet. The expansion velocity estimated from the experimental data was consistent with the simulation. In addition, we measured the penetration depth of neon ice pellets into the high temperature plasma, which provideduseful input for validating the theory and simulation codes that have been used to evaluate the ITER SPI system.
This research result was published on March 30, 2022 in Plasma and Fusion Research, an online journal by the Japan Society of Plasma Science and Nuclear Fusion Research. With the success of the first experiment that was performed in 2019, we conducted a second experiment that injected pellets made of a mixture of hydrogen and neon in the 23rd cycle in 2021. We are looking forward to obtaining new findings and interesting analysis from the new data.