Simulation analysis for boron coating on plasma facing surfaces using an impurity powder dropper
In the Large Helical Device (LHD), high-performance plasma discharges have been achieved with boron coating on plasma facing surfaces by boron powder injection into the plasma using an impurity powder dropper (IPD). The boron transport in this case was analyzed by simulation. It revealed that while the injected boron was deposited at positions far from the IPD for lower plasma densities, the boron was also locally deposited at positions near the IPD for higher plasma densities. The simulations provide a useful guideline for making effective use of the IPD.
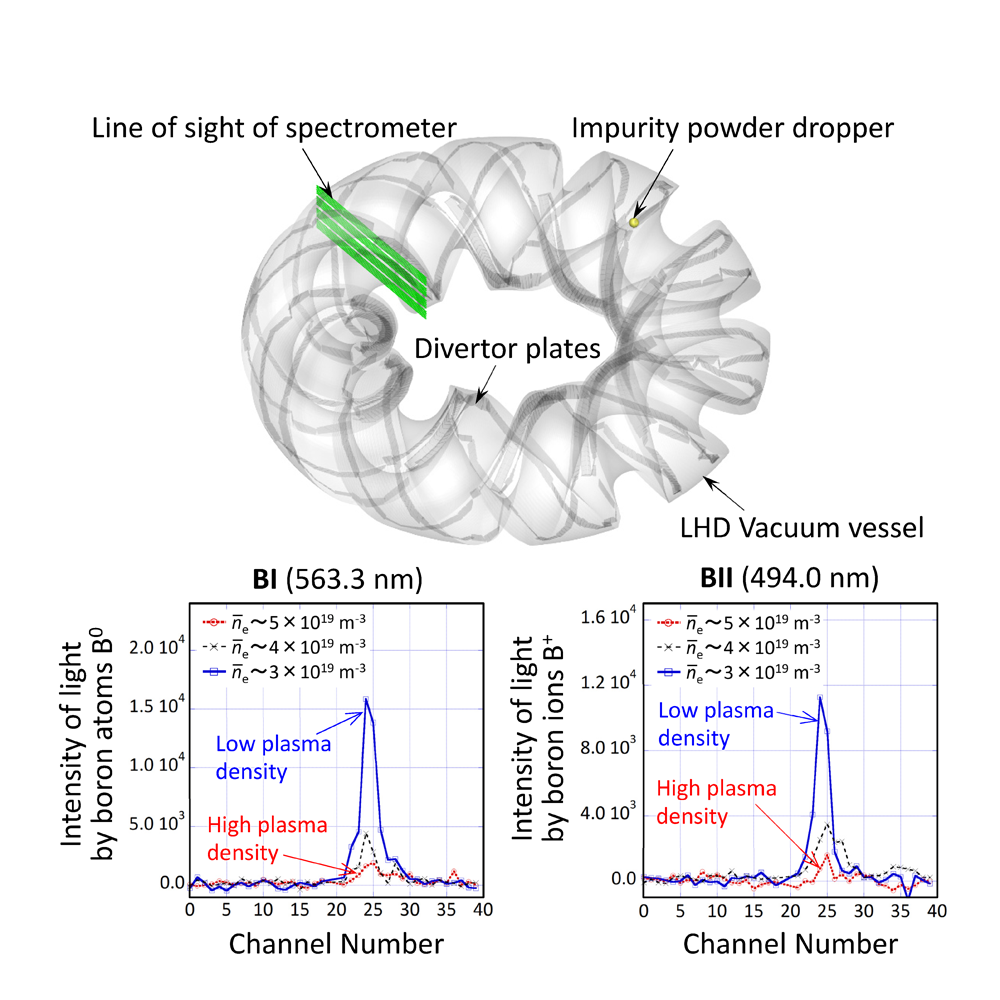
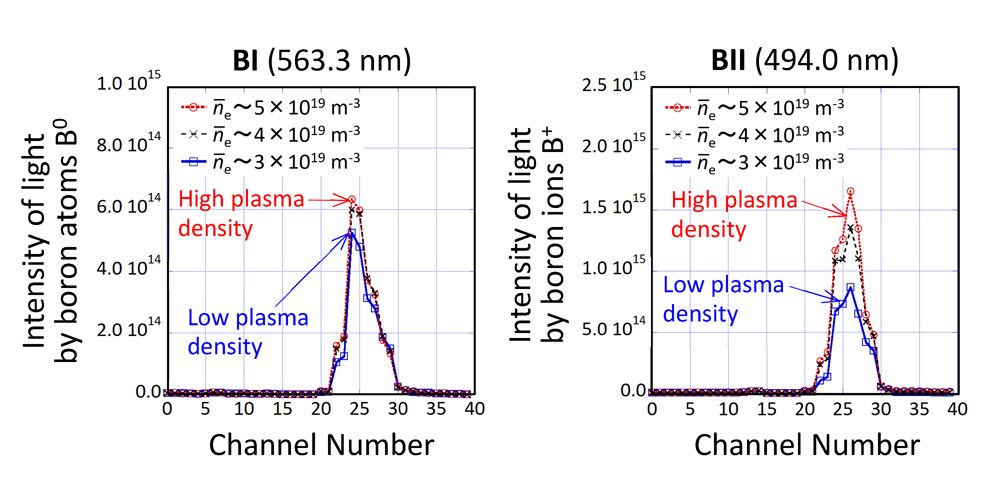
Sustaining steady-state high-temperature and high-density plasmas is essential for nuclear fusion reactors. It has been recognized that conditioning the surface on plasma-facing components on which the plasma directly touches (wall conditioning) is a critical issue. In the Large Helical Device (LHD), an Impurity Powder Dropper (IPD) was installed under a framework of international collaboration with the Princeton Plasma Physics Laboratory (PPPL) for effective wall conditioning. The IPD has a unique advantage over conventional wall-conditioning techniques, such as having no need to interrupt plasma discharge experiments.
Wall conditioning is performed by injecting small (about 0.1 mm in diameter) solid impurity (such as boron) powders into the plasma from the IPD. The solid impurity powders injected by the IPD reach the LHD peripheral plasma, by which the temperature of the powders rises due to the heat load from the plasma. When the temperature reaches the boiling point, impurity atoms are released from the powders into the plasma. The impurity atoms are ionized in the plasma to produce impurity ions which move along magnetic field lines for plasma confinement. For effective wall conditioning using the IPD, it is necessary to deliver the impurity ions onto the surfaces of the plasma-facing components and to deposit the impurities on the surfaces. Toroidally uniform deposition of the impurities on the surfaces of the plasma-facing components in the plasma-confinement devices can be advantageous for effective wall conditioning. The IPD was installed at one toroidal position in the upper part of LHD, which does not guarantee a toroidally uniform deposition of the impurities on the plasma-facing components. For this reason, an experimental condition for realizing the toroidally uniform deposition of the impurities was investigated using dust transport simulation. It revealed that impurity (boron) powder injection in lower-density plasmas achieves toroidally more uniform impurity deposition compared to that in high-density plasmas.
To verify the simulations, an experiment was performed by injecting boron powders into plasmas with various densities. In this experiment, the intensity profiles of boron emissions were measured with a multi-channel spectrometer installed far from the IPD. Figure 1 shows the measurements in this experiment, in which the intensity profiles of the emissions from boron atoms and singly charged boron ions are indicated in the upper and lower figures, respectively. In this figure, the intensity profiles were obtained by subtracting the intensity profiles before the boron powder injection from those during the injection. The measurements show that while the intensity around channel 24 is high during the boron powder injection for lower plasma densities, it is low for higher plasma densities. The line of sight of this channel crosses the surface of the plasma-facing wall (divertor plate) in the inboard side of the torus. Thus, the measurements suggest that the density of boron deposited on the surface of the divertor plate installed far from the IPD is low for higher plasma densities. Next, An analysis using plasma-wall interaction simulation was performed. In this simulation, the surfaces on the divertor plates inside the vacuum vessel were uniformly covered with boron. Figure 2 shows the calculated intensity profiles of the emissions of boron obtained by the simulation for three plasma densities, in which only the influence of the boron powders injected by the IPD is included. The simulation demonstrates that the intensity of the boron emissions increases with the plasma density. It is inconsistent with the measurements, which indicates that the assumption in the simulation ‘the surfaces on the divertor plates inside the vacuum vessel are uniformly covered with boron’ is not valid for higher plasma densities. This supports the above-mentioned result by the dust transport simulation (impurities dropped from the IPD deposit in the vacuum vessel more uniformly for lower plasma densities compared to that for higher plasma densities). This result provides a useful guideline for making full use of the IPD for effective wall conditioning.
This research was done as an international collaboration research activity by Mamoru Shoji at the National Institute for Fusion Science, Gakushi Kawamura at the National Institutes for Quantum Science and Technology, Roman Smirnov at the University of California, San Diego, USA, and Dr. Juri Romazanov et al. at the Forschungszentrum Jülich, Germany.
This research result was published in Nuclear Materials and Energy, a journal on material application in the production of nuclear energy, on November 7, 2024.