First observation of degradation of confinement and reversal of plasma flow due to broken confinement magnetic field
In the Large Helical Device (LHD), the leakage of the plasma particles and the reversal of the plasma flow due to the broken confinement magnetic field are observed. The broken confinement magnetic field is produced by changing the direction of the plasma current which is driven by the electromagnetic wave. These findings show that the direction of the plasma current driven by the electromagnetic wave is important for the plasma confinement and the braking of the plasma flow, which was observed in the previous research, is caused by the reversed force due to the breaking magnetic field.
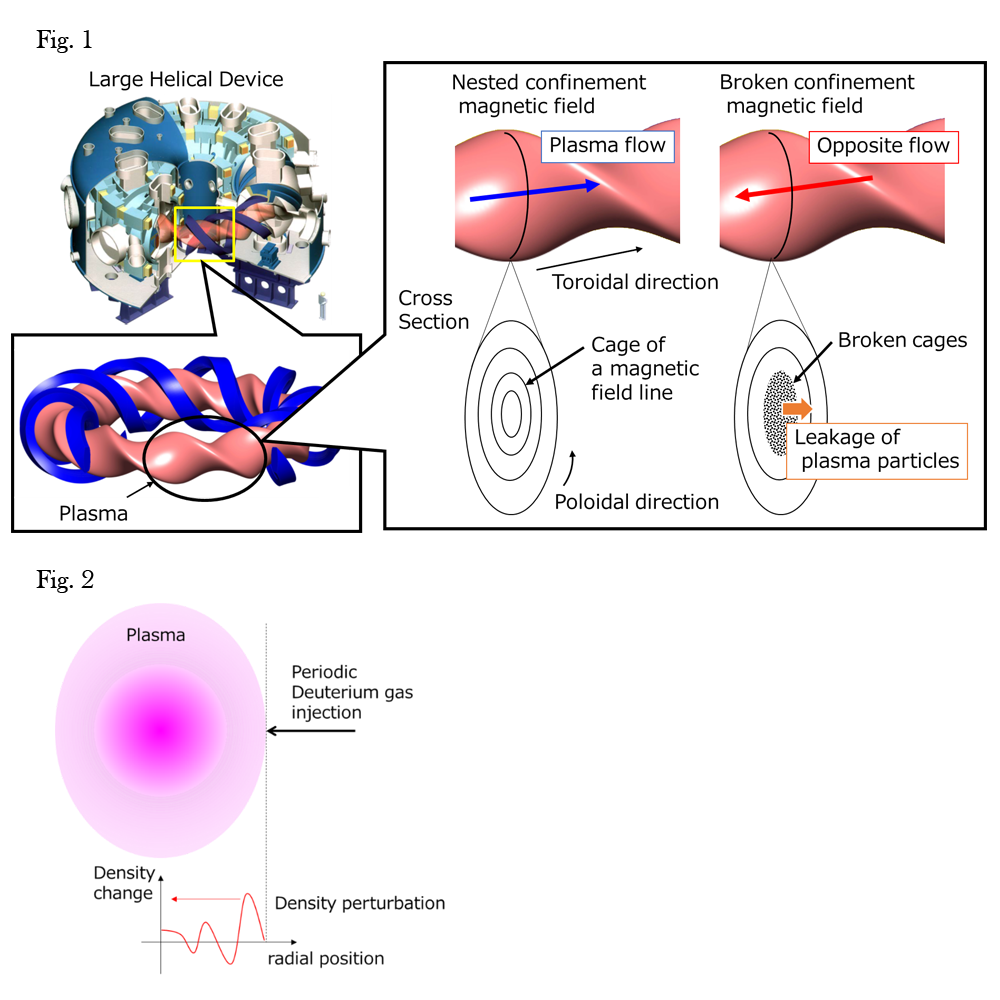
Fig.2. Periodic deuterium gas injection induces density perturbation in the peripheral region. By measuring the penetration of the perturbation into the core, the confinement of the plasma particles is evaluated.
High-temperature and high-density plasma should be maintained in the fusion reactor vessel with a high confinement state. Since the plasma particles are charged and follow the magnetic field lines in a helical motion, the plasma can be confined by nested magnetic field cages. However, when the temperature and the density become too high, the confinement magnetic field cannot suppress the plasma pressure depending on it [the temperature × the density], and the plasma becomes unstable. Therefore, it is necessary to know the mechanisms for the particle supply and exhaust control of the plasma density. Furthermore, since the fusion reaction takes place in the center of the plasma, it is important to know how to control the state of the plasma core from the outside.
We investigated whether it was possible to control particle exhaustion by changing the structure of the magnetic cage in the plasma core. To achieve this, we used electromagnetic wave injection, which is one of the plasma heating methods (electron cyclotron resonant heating). In electron cyclotron resonant heating, an electromagnetic wave with a frequency which corresponds to the gyrofrequency, is injected. This electromagnetic wave can selectively heat the electrons in the plasma and provide kinetic energy to them. By changing the direction of the electromagnetic wave injection, a plasma current can be generated in the core. The current generates the magnetic field in the circumferential direction of the plasma cross-section (poloidal direction: see Fig. 1.). Thus, it is possible to change the torsion, which is the number of times the magnetic field lines turn in the direction of the doughnut circle (toroidal direction: see Fig. 1.) during one round in the poloidal direction. The magnetic field line tracing code shows that a broken magnetic field cage in the core can be created when this torsion reaches a specific state.
We compared the confinement of the plasma with a magnetic field cage up to the core, with one having a broken magnetic field cage at the core. Deuterium gas, the plasma fuel, was periodically injected into the plasma. Then we measured how the density fluctuations generated at the periphery, penetrated the interior. If the fluctuations hardly propagate to the plasma core, the plasma is well confined, and the particles hardly leak out. When a broken magnetic field cage is present in the core, the fluctuations propagate very quickly only in that region. The particle leakage is about 20 times larger than when the magnetic field cage is present.
In addition, we compared the plasma flow in the toroidal direction with and without the magnetic cage. As a result, we found that the plasma flow in the broken magnetic cage becomes opposite to that in the magnetic cage. In the previous study, toroidal flow was braked when the broken magnetic cage existed. However, it was unclear whether this phenomenon was caused by the momentum transport from the fast flow to the slow flow, or by the force generated by the breakdown of the magnetic field cage. The electromagnetic waves do not drive the plasma flow in the toroidal direction, and the flow in the broken magnetic field cage is opposite to that in the nested cage. Thus, we concluded that the brake was caused by the force generated by the breakdown of the magnetic field cage. In order to understand how the force is generated from the broken magnetic cage, a more detailed investigation is needed.
Since fusion plasmas are expected to be larger than those in existing experimental reactors, it will not be easy to control the plasma characteristics in the core region directly. Therefore, since the plasma state in the core region is a result of the control of the peripheral region, it is necessary to obtain a knob for direct control of the core region. We have obtained important knowledge that the confinement characteristics and the plasma flow can be controlled only in the core, by controlling the magnetic field structure with the plasma current generated by electromagnetic waves.
This research was led by Yoshiaki Ohtani of National Institutes for Quantum Science and Technology (QST) with the cooperation of a research group led by Kenji Tanaka of the National Institute for Fusion Science (NIFS) and Yasuhiro Suzuki of NIFS (currently Hiroshima University), Mike Sanders of Eindhoven University of Technology, and Takahiro Bando of QST (currently Toyohashi University of Technology).
This research result was published on February 12, 2021 in Nuclear Fusion, a journal on controlled thermonuclear fusion by the International Atomic Energy Agency.