Successfully reproduced behavior of solid impurity dust particles in plasmas by numerical simulation
The melting and ablation processes of solid (dust) particles, including impurities, were observed when the dust particles were dropped into plasma in the Large Helical Device (LHD). Accurate prediction of the ablation position is an important issue because the ablation of the dust particles produces an impurity source in the plasma. The ablation positions were calculated using both dust particle transport and edge plasma simulation codes, which successfully reproduced the observations.
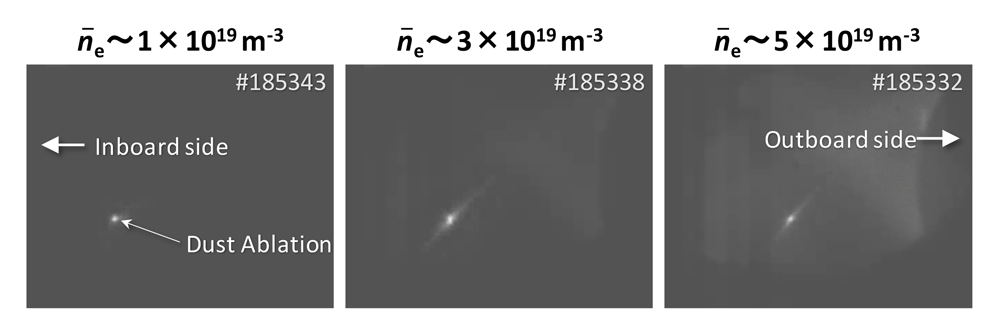
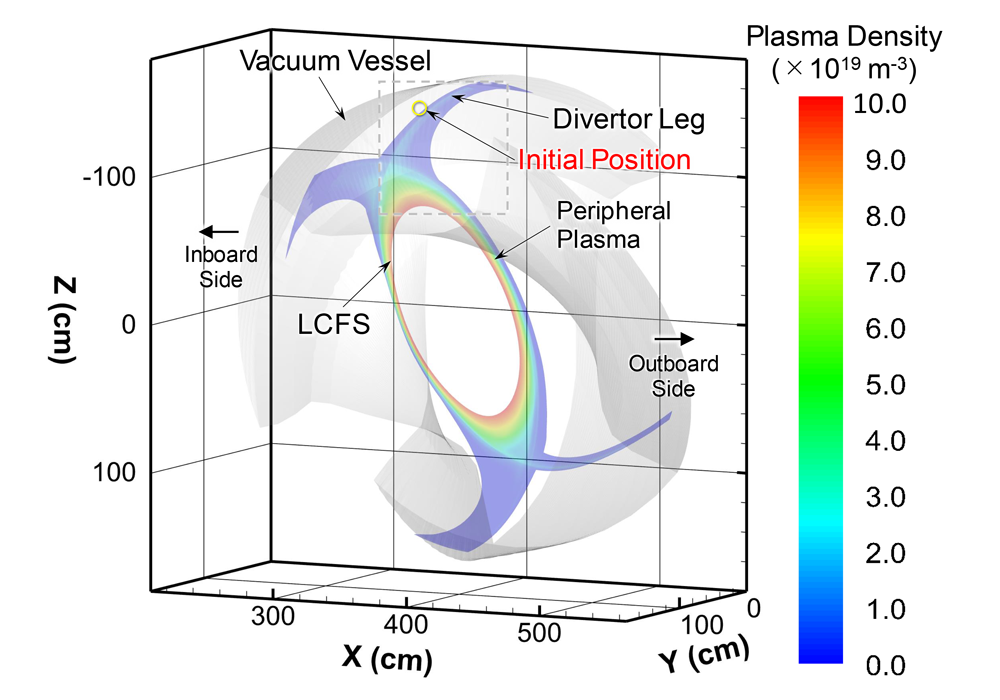
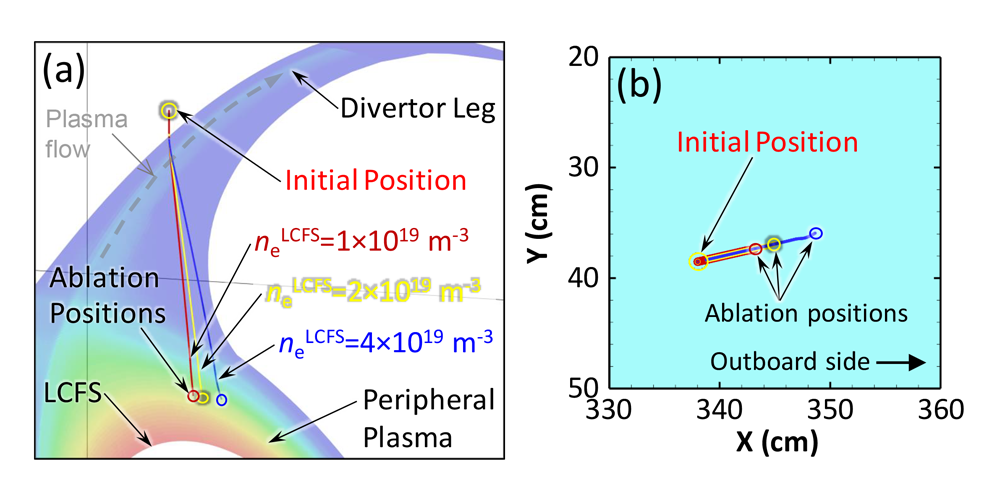
In the Large Helical Device (LHD), an Impurity Particle Dropper (IPD) has been used to inject small solid impurity (dust) particles into the plasma. For example, it was found that the injection of boron dust particles formed boron deposition layers on the surface of the vacuum vessel, the divertor plates, etc. (boronization), which successfully improved energy confinement in the plasma. When the dust particles are injected into the plasma, they are melted by the plasma heat load, which results in their ablation and disappearance with the emission of visible light. Since the ablated impurity atoms produce impurity sources in the plasma, it is a very important issue to observe and predict the ablation position of the dust particles.
The ablation positions in plasmas have been routinely observed with a fast-framing camera mounted at a quartz viewport at an upper port near the IPD. Figure 1 presents an image of the ablation of boron dust particles observed with the fast-framing camera. The left, middle, and right images show the observations in low, medium, and high-density plasmas, respectively. The ablation position changes to the right side on the images (corresponding to the outboard side of the torus) with the increase in the plasma density. The changes in the ablation position, depending on the plasma conditions, prevent control of the plasmas in experiments using the IPD. It is therefore important to clarify the reason for the change in the ablation position.
In this study, a prediction of the ablation positions of boron dust particles was tried using both a dust transport simulation code (DUSTT) and an edge plasma simulation code (EMC3-EIRENE). Figure 2 presents a three-dimensional model of the LHD vacuum vessel (for a toroidal angle of 18 degrees) for this analysis. The cross-section of a typical plasma density profile is shown at the toroidal angle where the dust particles drop in this model. Boron dust particles were dropped from just above an upper diverter leg (the yellow circle in this figure). First, a three-dimensional profile of the plasma parameters (density, temperature, etc.) was calculated using EMC3-EIRENE under different operational conditions. Next, the trajectories of the dropped boron dust particles were calculated using DUSTT. Then the ablation positions, where the dust particles evaporated and disappeared due to the heat load from the plasma, were obtained.
Figure 3(a) is a perspective view (corresponding to the area surrounded by the broken gray square in Figure 2), showing the calculated trajectories and the ablation positions of the boron dust particles for three different plasma densities (1, 2, 4×1019 m-3 at the Last Closed Flux Surface (LCFS)). Figure 3(b) presents these calculations viewed from the upper side. The ablation position changes to the outboard side of the torus with the increase in the plasma density, which is in qualitative agreement with the observations shown in Figure 1. Consequently, the simulation reveals the reason for the change in the ablation position with the increase in the plasma density.
Dust particles dropped from the IPD have to cross an upper divertor leg before reaching peripheral plasma. In the divertor leg, the velocity of the plasma flow is high, and the direction is towards the outboard side of the torus, which causes a deflection of the trajectory of the dust particles to the outboard side. This effect increases with plasma density. Therefore, the higher the plasma density is, the more the trajectory of the dust particles is deflected to the outboard side, which results in the change of the ablation position to the outboard side.
We have successfully reproduced the observations of dust particle behavior in LHD plasmas by the simulation. Using this analysis, the position of impurity sources in the plasma can be predicted in advance of the experiments. This enables us to make more effective use of the IPD and contribute to further improvement of plasma confinement.
This research was conducted in collaboration with Mamoru Shoji and Gakushi Kawamura of the National Institute for Fusion Science, Roman Smirnov of the University of California, and Yasunori Tanaka of Kanazawa University.
The results of this research were published in Journal of Advanced Simulation in Science and Engineering, a journal of the Japan Simulation Society, on 6th February 2024.